Glutathione, which is abundant in many bacteria, is known to bind to copper and has long been thought to help bacteria process copper.
Copper is an essential metal for bacterial physiology, but excessive copper is toxic to bacteria.
To limit copper levels in the cytoplasm, most bacteria have transcriptional response systems for copper output.
In the Gram-positive human pathogen Streptococcus pyogenes (group A Streptococcus [GAS]), the system is encoded by copYAZ operons.
In this paper, the author took Streptococcus pyogenes as the object of study and constructed mutant strains lacking copper efflux pump copA.
The role of glutathione in Streptococcus pyogenes was explored and it was demonstrated that glutathione increased the threshold of intracellular copper availability tolerated by bacteria, advancing the basic understanding of bacterial copper treatment.
First, in order to determine whether the Cop system and its interaction with host Cu have an effect on the pathogenesis of GAS, the authors established an aggressive disease model using transgenic human plasminogen mice.
Mice subcutaneously infected with wild-type GAS developed ulcerative skin lesions at the injection site 1 day later.
These lesions were removed 3 days after infection and were found to contain more Cu than adjacent healthy skin or the skin of uninfected mice.
The mice also had increased levels of Cu in their blood three days after infection.
The authors also compared the survival rate of infected mice, and found that there was no statistical difference between infected wild-type and copA mutant mice, and ΔcopA mutant was not more likely to be killed by human neutrophils than wild-type or copA mutant mice.
These results suggest that despite the elevated systemic and niche specific levels of host Cu,
the Cu efflux pump CopA not required for GAS virulence in this model.
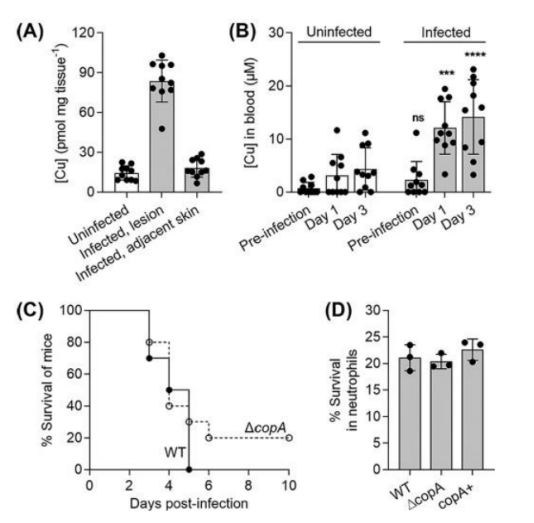
Due to the lack of virulence defects of copA in vivo, the authors further investigated the effects of Cu treatment on GAS physiology.
It found that adding Cu(up to 10 M) to the medium did not affect the doubling time of ΔcopA mutants during the exponential growth phase, but it did reduce the final culture yield.
At the same time, in each condition, regardless of growth rate,
the copper-treated cultures stopped growing when they reached approximately the same optical density,
indicating that the growth defect was related to the number of bacterial cells and/or the stage of growth.
Parallel evaluations of plating efficiency and total ATP levels confirmed that differences between Cu-treated and untreated cultures found only in the exponential late or early stable stages of growth,
during which Cu-treated copA cultures showed a significant decrease in plating efficiency and ATP production compared to untreated controls.
Therefore, the authors conclude that Cu treatment leads to defects in the later exponential stage of growth.
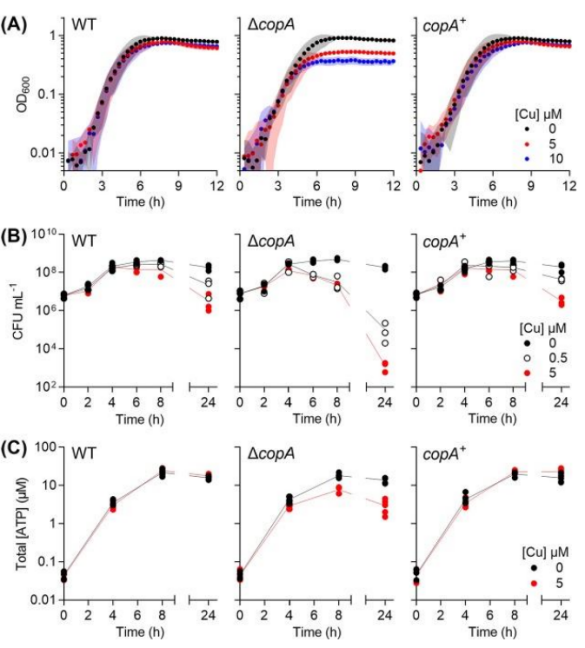
GAS is a kind of lactic acid bacteria.
Under our experimental conditions, this microbe undergoes homolactic fermentation, producing lactic acid as the main end product.
However, Cu-treated copA cultures did not acidify the growth medium,
so the authors hypothesized that Cu treatment impaired fermentation in GAS.
The authors found that Cu-treated ΔcopA cultures produced 50% less lactic acid and consumed 50% less glucose than untreated controls,
and the differences only emerged after 4h.
The authors also found that Cu-treated copA cultures did not consume glucose after 4 hours.
So these results suggest that Cu treatment leads to metabolic defects,
but only after entering the later exponential phase of growth.
The authors suggest that the loss of lactic acid production, rather than pyruvate production, implies lactate dehydrogenase (Ldh) inactivation.
To validate this suggestion, we cultured GAS for 4 hours in the absence or presence of added Cu,
prepared whole cell extracts, and measured Ldh activity.
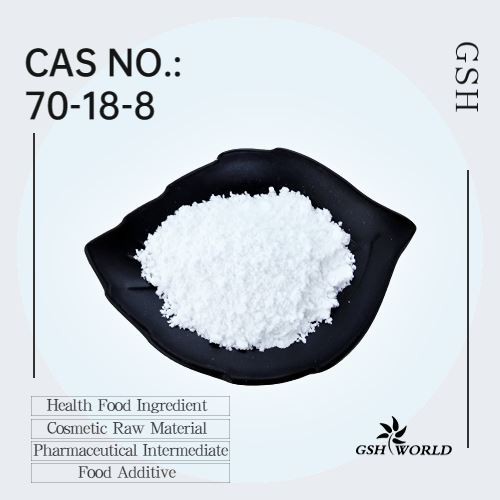
However, Ldh remained active in all strains regardless of Cu treatment.
To find the target of GAS copper poisoning, the authors studied GAPDH(glyceraldehyde-3-phosphate dehydrogenase) enzymes in GAS,
namely classical, phosphorylated, ATP-producing GapA and alternative, non-phosphorylated GapN.
As expected, Cu treatment led to a reduction in GapA activity in ΔcopA mutant cells,
which could explain the decrease in lactic acid secretion and ATP production.
Reduced GapA activity also leads to the accumulation of upstream glycolytic precursors,
which leads to feedback inhibition of downstream enzymes (40), as well as glucose phosphorylation and uptake.
At the same time, when cultures sampled earlier (at 1/2 and 3 hours),
no differences observed between the GapA activity of Cu-treated cells and control ΔcopA cells.
The timing of GapA inhibition at the beginning of the exponential phase of late growth coincides with the stagnation of bacterial growth and metabolism,
supporting the hypothesis that GapA is a key target for Cu poisoning in GAS.
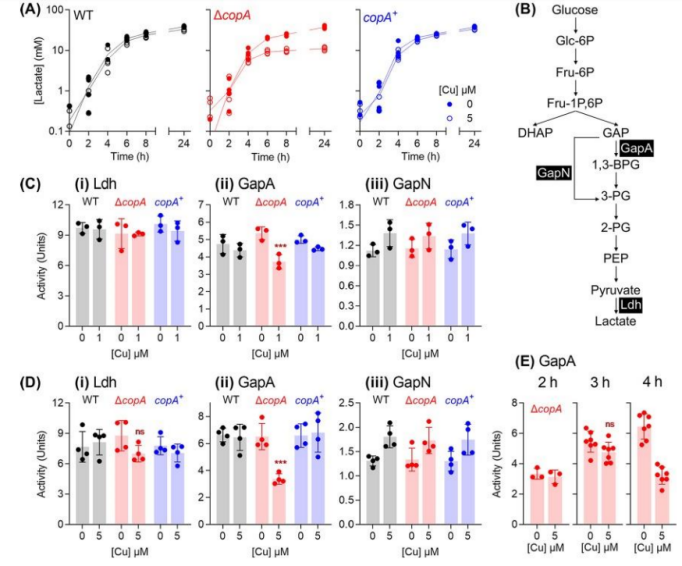
To verify the protective effect of glutathione,
total intracellular copper levels measured in ΔCopA mutant cultures supplemented with copper and/or glutathione.
As expected, when ΔCopA cultures challenged by low concentrations of added copper (500nM), glutathione supplementation did not inhibit total intracellular copper levels.
And at high concentrations of added copper (5M),
glutathione-filled cultures appeared to accumulate higher intracellular copper levels, rather than lower copper levels.
However, these cultures did not exhibit a significant copper stress phenotype.
These results suggest that the protective effect of glutathione is not related to the chelation of extracellular copper ions or the reduction of intracellular copper uptake.
Moreover, it found that the intracellular Glutathione levels of both strains decreased to 0.1mM at t4 h regardless of Cu treatment.
This decline may caused by bacteria growing and repliating in a chemically defined medium with limited supply of Glutathione and low intracellular levels of Glutathione consistent with the onset of the observed Cu-stressed phenotype.
As mentioned earlier, these GSH-treated cells are copper tolerant.
In fact, these cells accumulated more Cu within their cells than controls that not treated with GSH.
A more detailed examination of GSH-supplemented ΔcopA cells confirmed that the addition of Cu protected GapA from inactivation.
In addition, Cu-induced time-dependent changes in cadD and adcAII expression eliminated, indicating that CadC and AdcR not mismetallized.
Overall, these results support a model that GSH constitutes a major buffer for excess intracellular Cu in GAS and protects potential non-homologous binding sites from Cu metallization.
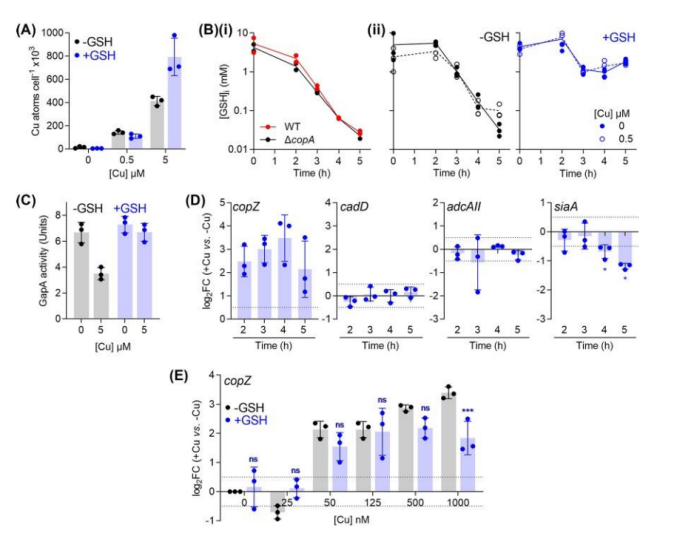
To sum up
The authors concluded that the inactivation of copA effector does not reduce the virulence of GAS.
In vitro, copper treatment causes a variety of observable phenotypes, including growth and viability deficits,
reduced fermentation, inhibition of glyceraldehyde-3-phosphate dehydrogenase (GapA) activity, and dyshomeostasis of metals,
as well as experimental results showing that extracellular GSH supplementation can replenish intracellular mercaptan pools and inhibit all observable effects of copper treatment.
These results further demonstrate that glutathione increases the threshold of intracellular copper availability tolerated by bacteria,
providing new evidence for the study of Group A streptococcus.